Optical magnetometry
Optical atomic magnetometry uses the relationship between the optical properties of a medium and the external magnetic field. In our experiments, rubidium atoms interact non-linearly with the resonant laser beam. This allows us to obtain optical properties that are extremely sensitive to the magnetic field. In these experiments, the necessary step is to create and maintain the coherence between Zeeman sublevels in atoms. Laser light is used to both generate the appropriate coherence and to probe the obtained anisotropy by measuring the polarization rotation angle of the beam propagating through the medium – the so-called non-linear Faraday effect. The strong dependence of the measurement result on the applied magnetic field allows, on the one hand, to construct a very sensitive magnetometer, and on the other hand, it enables the controlled manipulation of atomic states – the so-called quantum state engineering.
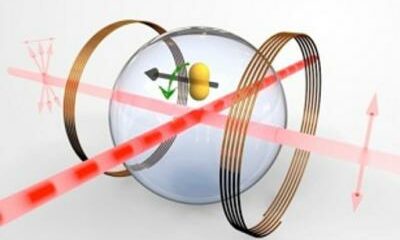
GNOME – Global Network of Optical Magnetometers for Exotic physics search
A novel experimental scheme enabling the investigation of transient exotic spin couplings is based on synchronous measurements of optical‐magnetometer signals from several devices operating in magnetically shielded environments in distant locations (≳ 100 km). Although signatures of such exotic couplings may be present in the signal from a single magnetometer, it would be challenging to distinguish them from noise. By analyzing the correlation between signals from multiple, geographically separated magnetometers, it is not only possible to identify the exotic transient but also to investigate its nature. The ability of the network to probe presently unconstrained physics beyond the Standard Model is examined by considering the spin coupling to stable topological defects (e.g., domain walls) of axion‐like fields. In the spirit of this research, a brief (∼2 hours) demonstration experiment involving two magnetometers located in Kraków and Berkeley (∼9000 km separation) was presented in the article published in 2013 and discussion of the data‐analysis approaches that may allow identification of transient signals was provided. Now, the GNOME collaboration is spanning world-wide.
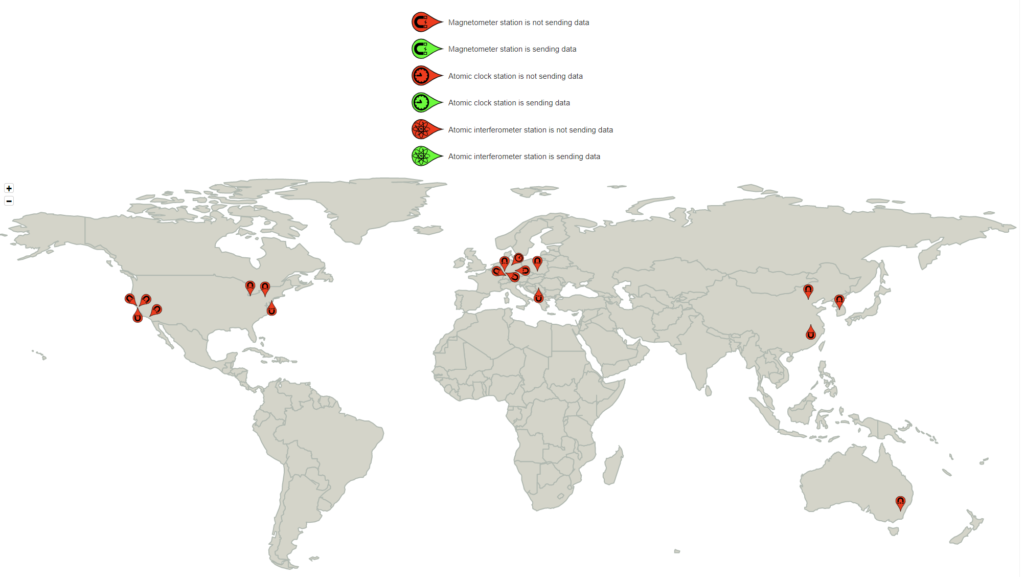