Optical Atomic Clocks
Optical Atomic Clocks’ group operates state-of-the art optical frequency standards, develops next generation passive and active systems, and, above all, use these wonderfull apparatus to study new physics.
POZA
The Polish Optical Atomic Clock (POZA) was constructed together by researchers from Jagiellonian University, University of Warsaw and Nicolaus Copernicus University in Toruń
POZA is a system of two independent strontium optical lattice clocks. The system consists of two atomic standards interrogated by a shared ultra-narrow laser, pre-stabilised to a high-Q optical cavity and an optical frequency comb
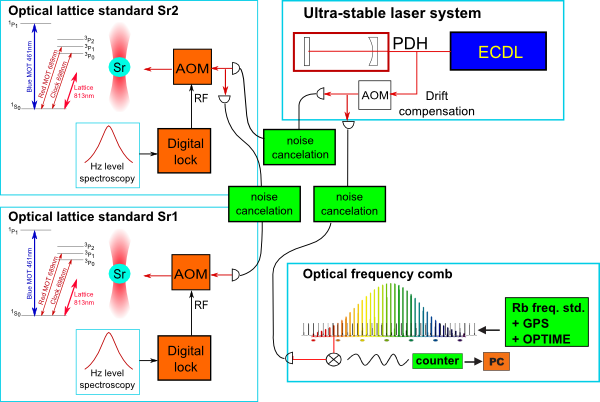
For the average times τ greater than 60 s the fractional stability of our clock increase with σy (τ ) = 3.41(27)×10−14/√(τ) and reach 2*10-15 after 5 minutes.
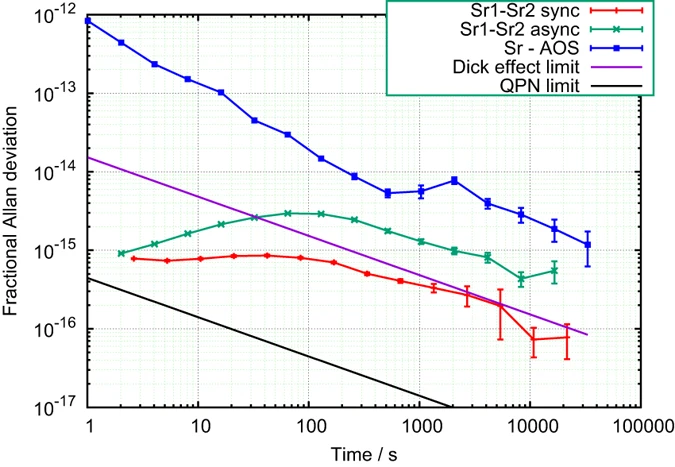
KL FAMO Laboratories are the first facilities connected through dedicated fibre optic network with noise elimination to transfer the standard time and frequency signal from POZA. It is also planned to transfer the time signal to other laboratories, which are now forced to use their own, less accurate frequency standards for research purposes. The plans are feasible due to the globally unique model of the nationwide network research infrastructure PIONIER, via the OPTIME network, combined with a good cooperation between the Consortium members and Orange Polska. Similar networks for the transfer of standard time and frequency signal exist in just a few countries worldwide.
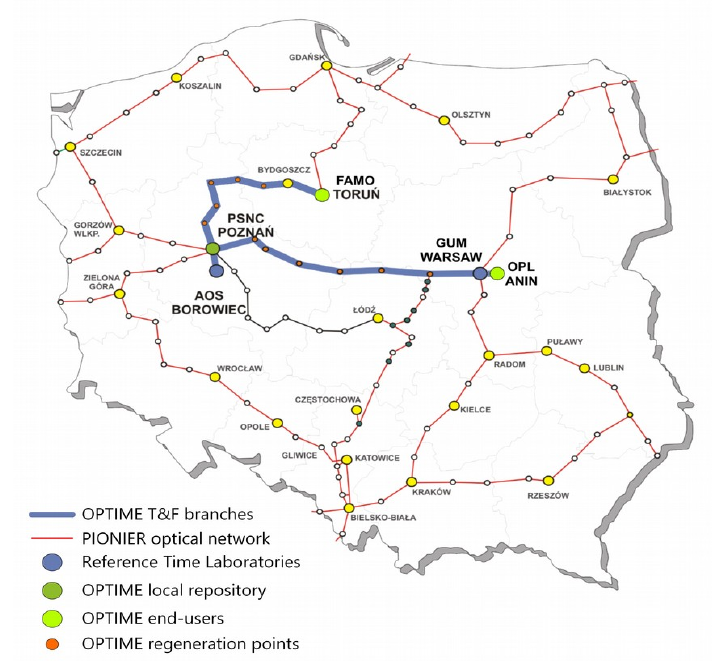
Active Optical Clock
An ordinary optical atomic clock of the present generation is a passive frequency standard. It consists of a laser locked to an optical cavity which periodically probes the frequency of atomic transition. Between each interrogation, new atomic sample is prepared. During each interrogation the phase of the laser is imprinted to the atomic sample, but then this information is lost in the new clock cycle. This determines how fast the stability of the clock can possibly increase as a function of measurement time.
In an active optical atomic clock photons are directly generated from atoms as a result of light emission on the clock transition. The light is in resonance with atomic clock transition at all times and at any time its phase does not deviate from the phase of atoms. Therefore, the achievable stability of an active optical clock is restricted by white phase noise and is much higher than for the ordinary passive optical clock operating on the same clock transition. In the microwave regime that is one of the reasons why active hydrogen masers are much more stable than passive active masers.
Thanks to the superradiance phenomenon, atoms themselves, not the optical cavity, can act as the clock’s flywheel. This can be achieved by cavity-induced long range coherence among the atomic dipoles which subsequently radiate coherently in the superradiant regime.
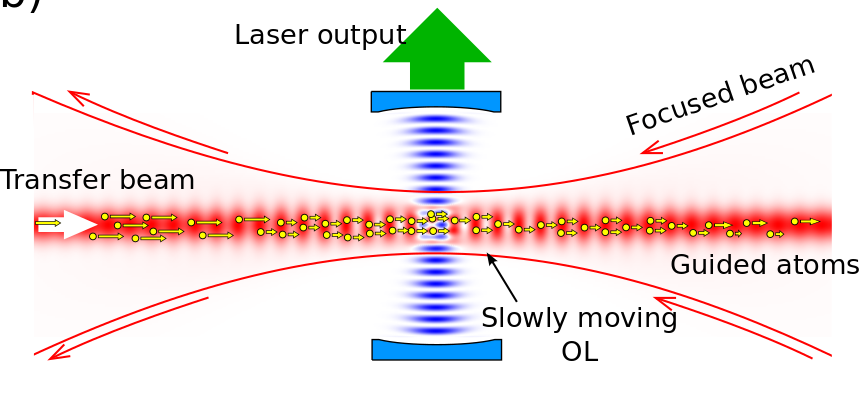
Within the H2020 Quantum Flahship project iqClock we are collaborating with the University of Amsterdam, The Vienna University of Technology, and University of Innsbruck groups to build a continuously operating superradiant frequency standard, operating on the Sr clock transition.
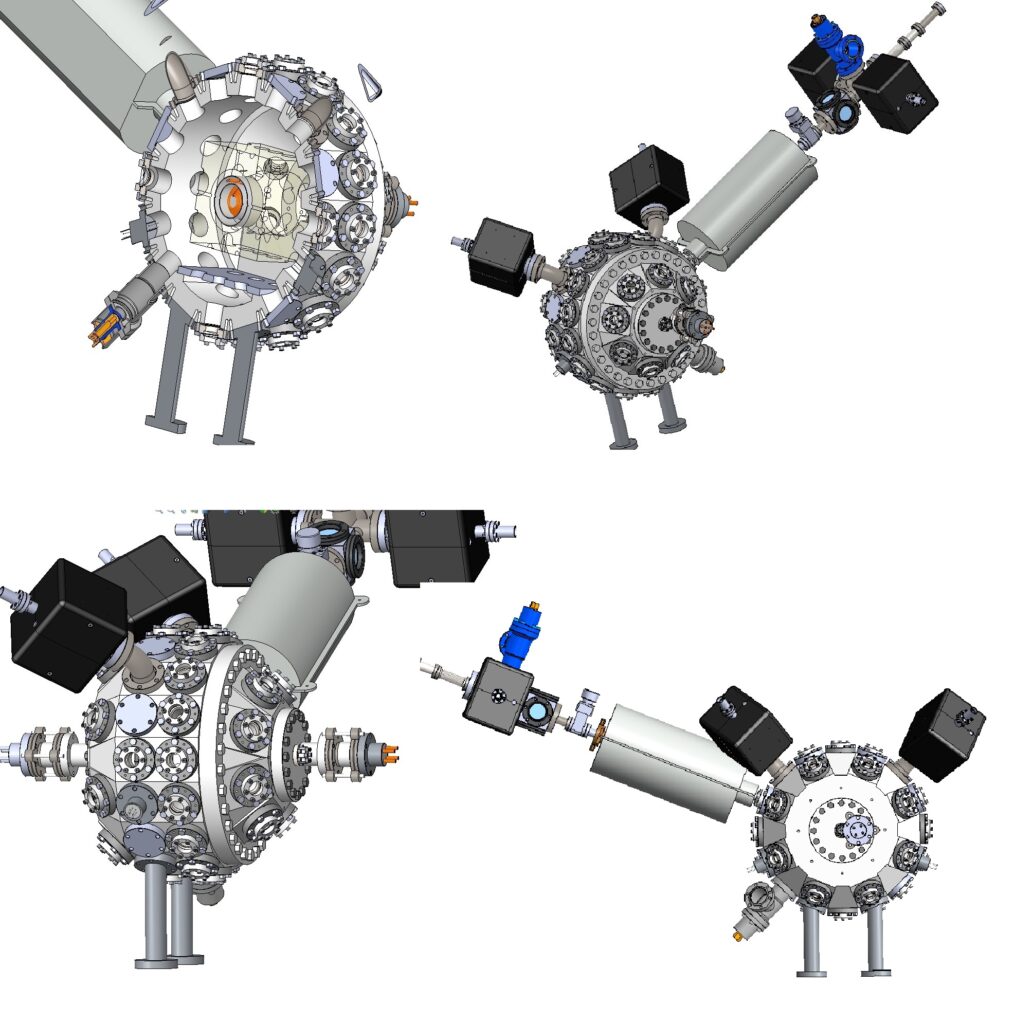
Fundamental physics and beyond
Optical atomic clocks are the most precise scientic instruments available to humanity. Their accuracy and stability reach eighteen signicant digits. Therefore, optical atomic clocks are one of those experiments that push the boundaries of knowledge and metrology. They are used as quantum sensors
and standards, inter alia, in relativistic geodesy, in radio astronomy, in search for variations of fundamental constants, validating assumptions of the Standard Model, and, as we showed recently, also in detection of dark matte. The cost of their construction and maintenance, however, is many times lower and the needed research team is smaller in comparison to other great experiments that enlarge our knowledge on structure and history of our Universe, and as a consequence, bring new emerging technologies into our lives.
The unique properties of these sensors are direct consequences of a typical optical atomic clock setup. A standard optical atomic clock consists of two state-of-the-art components: an ultra-stable high-Q optical cavity which transfers stability of the length into stability of the frequency, and an atomic sample which transfers accuracy of the energy of the ultra-precise atomic clock transition into accuracy of the frequency. These two components have dierent susceptibilities to the external perturbations such as electric and magnetic elds, and to the possible changes of fundamental physical constants.
In our project we have established, with our scientic partners, the first earth-scale quantum sensor network based on optical atomic clocks with the coordination and research centre located at KL FAMO in Torun. The network of such detectors can be used as a state-of-the-art global sensor in a wide range of sectors from applications in geodesy, satellite navigation and environmental monitoring, including monitoring changes in ocean currents, oil and gas surveying, monitoring environmental changes such as
melting of the polar ice caps and volcanic processes that take place before an eruption, to basic science and metrology.
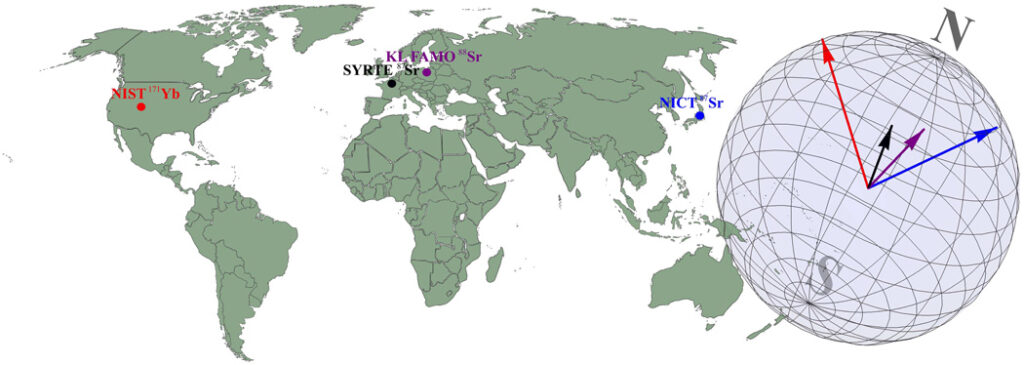